pion
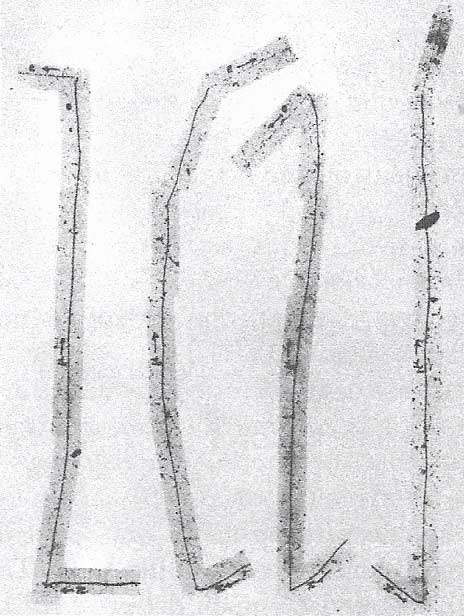
Figure 1. Some early examples of pion decays observed in a photographic emulsion in 1947. The positive pions come to rest before decaying by the reaction π+ → μ+ + νμ, to give monoenergetic muons. These travel about 600 mm in the emulsion before coming to rest and decaying by the reaction μ+ → e+ + νe + ν̅μ to give a positron.
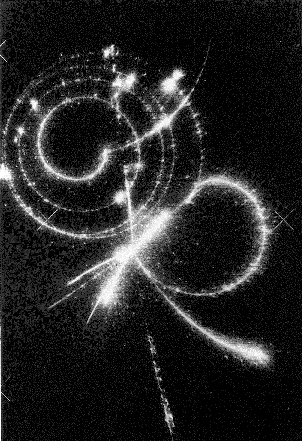
Figure 2. In this image from a streamer chamber, one of the pions from a collision makes the looping track to the right, before it decays into a muon, which then curls anticlockwise four times, and eventually changes into an electron which moves off towards the upper right. Image credit: Tom Kemp.
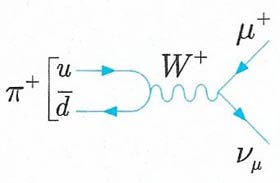
Figure 3. Quark diagram for the decay π + → μ + + νμ.
The pion is the lightest type of meson. Pions, also called pi-mesons, are composed of up quarks, down quarks, and their anti-quark counterparts. Pions of charge +1, -1, and 0 are denoted π +, π –, and π 0, respectively. The π 0 (mass 135 MeV) is composed of either an up/antiup quark pair or a down/antidown quark pair; the π + is an up/antidown pair, and the π – is a down/antiup pair (both have a mass of 140 MeV). All have zero spin.
Pions were predicted theoretically by Hideki Yukawa in 1935, and were discovered in cosmic ray experiments on the Pic du Midi by researchers from Bristol University, England, headed by Cecil Powell, in 1947. They are produced copiously in many high-energy particle collisions, specifically in hadronic reactions that conserve both charge and baryon number, for example in the proton-proton collisions
![]() |
The charged pions decay predominantly by the reactions
![]() |
with lifetimes of 2.6 × 10–8 second, typical of weak interactions. They were first discovered in cosmic rays by a Bristol group in 1947 using photographic emulsions containing a silver halide. The ionization energy deposited by a particle passing through the emulsion causes the formation of a latent image, and the silver grains resulting from subsequent development form a visual record of the path of the particle. As in a cloud chamber, the range of a stopping particle can be used to estimate its energy. Some historic examples of stopping π + decays observed in this way are shown in Figure 1. Because these are two-body decays, the muons in the final state all have the same energy. Consequently, they all travel approximately the same distance before coming to rest and decaying to give a positron. Neutral pions were discovered somewhat later and decay by the electromagnetic interaction
![]() |
with a lifetime of 0.8 × 10–16 second. Because they are neutral they do not leave tracks and must be detected via their decay photons.
Pions and nucleons are the lightest known mesons and baryons, respectively, and are bound states of the lightest quarks (u, d) and their antiquarks (u̅, d̅). The only 3q and qq̅ combinations that give the observed electric charges are then
![]() |
for the nucleons and
![]() |
for the pions. In π + decay, whose quark interpretation is
![]() |
and is shown in Figure 3, a u quark annihilates with a d̅ antiquark, demonstrating again that individual quark numbers like Nu and Nd are not conserved in weak interactions.
Pions play an important role in nuclear forces. In 1935 Yukawa proposed that these were due to the exchange of spin-0 mesons and from the range of the forces (which was not precisely known at that time) predicted that these mesons should have a mass of approximately 200 MeV/c2. The discovery of pions was a great triumph for the Yukawa theory. In it, the nuclear forces are given by the following model,
![]() |
The Yukawa model for nuclear forces: direct forces (upper three diagrams); exchange forces (lower two diagrams). |
where the nucleons and pions are treated as point particles. Neutral pion exchange gives rise to normal direct forces, while π ± exchange gives rise to "exchange forces" whereby the neutron and proton are interchanged. The resulting potential is of the general Yukawa type, and reproduces the longest range part of the nuclear force r ≥ 2 femtometer (fm) very accurately, including spin effects when these are taken into account. At short ranges r ≤ 1 fm, comparable with the sizes of the nucleons and pions themselves, this agreement breaks down completely. At these distances, the internal quark structure of the hadrons can no longer be neglected and the situation is much more complicated.
Finally, we note that atmospheric neutrinos are produced almost entirely from pion decays. When cosmic ray protons collide with atoms in the upper atmosphere, they create many charged pions in reactions. These decay almost entirely, followed by muon decay to give two muon neutrinos or antineutrinos for each electron neutrino or antineutrino.