FROM GLASSES TO GASES: The Science of Matter - 5. Str-e--t----c------h!
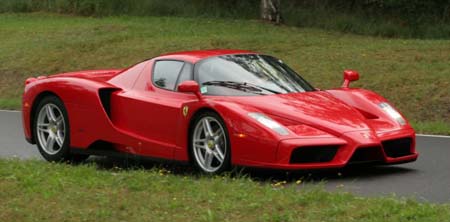
Figure 1. The tires and suspension of a car are elastic.
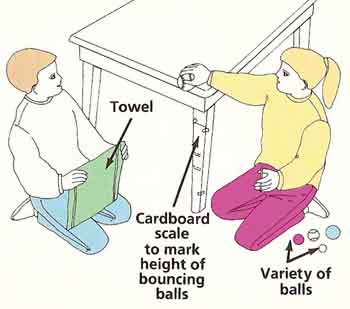
Figure 2. Rebound experiment.
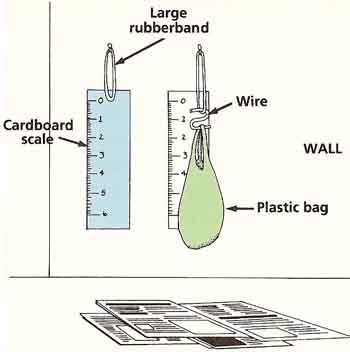
Figure 3. Stretching experiment.
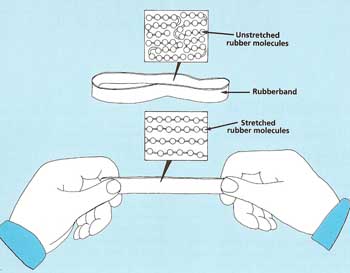
Figure 4. Molecules in a rubber band.
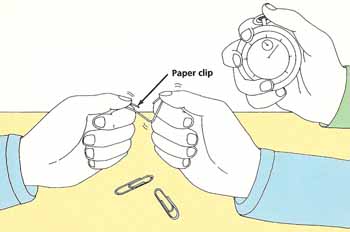
Figure 5. Paper clip test.
It would be a hard life without ELASTICITY. This is the property of substances that makes them bounce back after they have been stretched out of shape. Rubber is highly elastic. That's why we use it for making tires, balls, rubber bands, the soles of shoes, and all sorts of other things that need to be springy. Metal coiled into elastic springs provides comfortable support in sofas and beds.
Clues to a Disaster
After the space shuttle Challenger exploded a minute after takeoff in 1986, an inquiry was set up to study the disaster. The accident happened because a special seal, known as an O-ring, had failed in one of the shuttle's big solid-rocket boosters (SRBs) and allowed fuel to leak out. But why had the O-ring failed?
One of the people taking part in the inquiry was a leading American scientist, Richard Feynman. He had heard that the shuttle had taken off after a very cold night at the launch site. Icicles still hung from the spacecraft as its engines fired. Feynman put a piece of O-ring material into his drinking glass of ice water. A few minutes later, when he took it out, the O-ring was stiff instead of elastic. In this condition, it could no longer work as an effective seal. Through a simple experiment, Feynman had found the cause of the disaster.
![]()
Bouncing BackYou will need:
What to do: Tape the strip of card to a leg of the table. Hold the tennis ball over the edge of the table so that the middle of the ball is level with the tabletop. Your friend should be kneeling down, ready to see roughly how high the ball bounces. Let the ball fall. Ask your friend to to hold the edge of the towel level at the height that the middle of the ball seemed to reach after its bounce. Let the ball fall again while your friend looks at eye level over the edge of the towel held at this height. If the middle of the ball reaches exactly the line of the towel after the bounce, mark this height on the card and label it "tennis ball." Otherwise, drop the ball again with the towel wither slightly higher or lower (see Figure 2).
When you think you have an accurate mark for the first ball, repeat the test with the other balls. Remember to write down which ball corresponds to which line on the card. Then weigh each ball on the scales. Make a chart of the height of the bounce compared to the balls' weights. What can you conclude? Do the heaviest balls always bounce the most? Which are the best bouncers? What are they made of? Are they solid or hollow?
Taking it further: Put the bounciest of the balls in the freezer for one hour. Remove the ball and, to see how high it bounces, quickly carry out the above test. Has its elasticity changed? If so, is the ball more or less elastic than when it was warm? |
Springy molecules
The force you have to exert to pull apart a rubber band is the same as the force with which the molecules in the rubber are trying to pull one another back together again. When you stretch a rubber band, you are dragging molecules farther apart. When you relax your pulling, the molecules move back together again.
An elastic substance is one whose molecules can be separated quite a long way and still return to their original position. Rubber and skin both have this property. Other materials, like string and soft plastics, are fairly elastic. Still other materials, such as stone and glass, have a very low elasticity.
![]()
Stretching to the LimitYou will need:
What to do:
(It would be a good idea to spread newspapers below the experiment area to avoid a mess.) Hang the rubber band from a strong nail securely fixed to the wall. Ask an adult for help with this. Mark a scale in tenths of an inch on the strip of card. Fix the card on the wall behind the band so that when the band is straightened (but not stretched) the bottom of it reaches to the zero reading. Bend the wire to make an S-shaped hook as shown. Weigh the hook and the plastic bag. Hang the hook from the rubber band and hang the bag from the hook (see Figure 3).
Read off the new length of the band and write this down next to the weight of the hook and bag. Measure out 1 ounce of sand on the scale and pour this into the bag. Again, make a note of the rubber band's length next to the total weight hanging from it. Continue adding sand, 1 ounce at a time, recording the new length and weight. Do this until either the bag is full or the rubber band breaks. Plot a graph.
Compare the two pieces of wood that you had marked with your name. Which has been worn the most? Can you explain your findings?
Taking it further:
Carry out this experiment again, but with a metal spring in place of the rubber band. What happens? Invent a theory to account for your observations.
If weights are put on the end of an elastic material, the material stretches steadily at first. That is, the material increases its length by equal amounts if equal weights are added. The English scientist Robert Hooke first discovered this in 1678, so it is known as HOOKE'S LAW.
However, beyond a certain point, called the ELASTIC LIMIT, the material starts to behave differently. If too much weight is put on the rubber band, it reaches a stage where it cannot stretch any farther. Its molecules, instead of being tangled and loose, are pulled as tight as they will go. Any more weight causes the rubber band to snap.
A metal spring or wire, if taken past the elastic limit, does the opposite of a rubber band. It starts to stretch more and more for each additional weight than it did when it was obeying Hooke's Law. Beyond the elastic limit, a spring becomes stretched out of shape and will not coil up again properly even if the weight is removed. A wire, if overloaded, starts to flow almost like a liquid. It goes into a plastic state. Eventually, if enough of a load is put on it, the wire develops a narrow neck and breaks. An experiment to study elasticity in a metal wire should only be dome in school with the help of a teacher. |
Inside Rubber
The reason why rubber can be stretched so much is that it is built up of long chains of molecules, most of which are folded like tangled ropes (see Figure 4). When the material is pulled, the chains straighten themselves out. They return to their original tangled shape after the stretching force is removed. If the rubber molecules are pulled too hard, though, they snap apart.
Many other materials, such as wood and silk, are built of chains of molecules. But, in most cases, strong links between the chains prevent them from curling back on themselves, so the material is not very elastic.
![]()
Breaking PointYou will need:
What to do:
Straighten out all the paper clips. Hold one and bend the middle of it backward and forward as quickly as you can. Count how many times you have to bend it before it snaps. Have your assistant time how long it took from the first bend to the moment of snapping (see Figure 5). Write down these results.
Take another paper clip and bend it backward and forward at a steady rate, but a little more slowly than the first time. Make sure you bend the clip by the same amount as the first clip and by the same amount each time. How many bends did it take to break? How long did it take?
Repeat these steps for several other clips. Each time, reduce the rate at which you bend the metal. Finally, bend a clip extremely slowly. Take several seconds over each bend. Plot your results as a graph, with the number of bends to breakage plotted on the vertical axis and the time taken per bend on the horizontal axis. What shape is your graph? What can you deduce from this?
Taking it further:
Bend another paper clip backward and forward a few times very quickly. Put the bent part to your lip. What do you feel? Look at the bent part through the magnifying glass. Is the appearance of the metal that has been bent different from the metal in the rest of the clip? Bend the clip until it snaps. Examine one of the broken ends under the microscope. Describe your observations and make a sketch of what you see.
Carry out similar experiments with other types and thicknesses of wire. Are the results different if you bend the wire in iced or in hot water? Try to explain your findings. Experiment with bending and breaking other types of material, such as plastic. |
Metal Fatigue
Metal parts that are repeatedly put under a lot of stress may eventually lose some of their strength. This loss of strength is called METAL FATIGUE. It can be recognized by the appearance of tiny cracks, called fatigue cracks, on the surface of the metal.
The wings and bodies of aircraft, for example, have to be thoroughly checked at regular intervals for signs of fatigue. Engineers go over vulnerable areas with special equipment, looking for the first telltale microscopic cracks. If these cracks were to go unnoticed, they could spread throughout a vital part of the plane and cause a major failure in flight. Finding ways to avoid metal fatigue is important for anyone who builds vehicles or other machinery with hard-working metal components.