diffraction
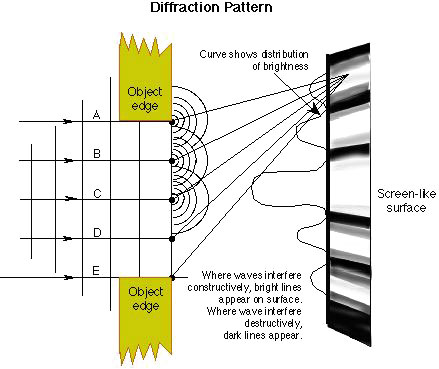
Diffraction pattern.
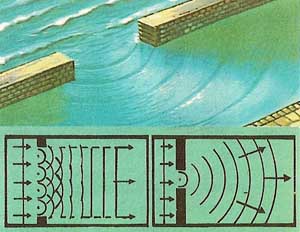
Water waves spread out from a narrow gap. The narrower the gap, the greater the proportion of diffracted waves. Huygen's construction (below) shows how the shape of the wave after diffraction can be found by drawing semicirculars around each point in the gap.
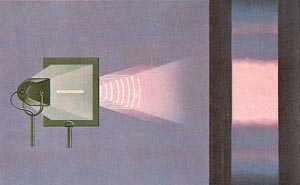
Light spreads out, or diffracts, from the narrow slit opening, to form a diffraction pattern on the screen.
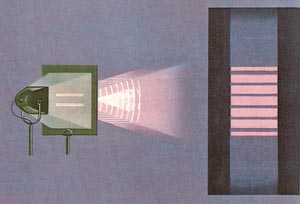
The diffracted beams from two slits interfere to make narrow bands of light and dark, interference fringes on the screen.
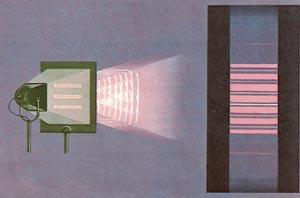
The effect of increasing the number of slits is to narrow and brighten the bright fringes. Smaller bright fringes between the major fringes disappear as more slits are used.
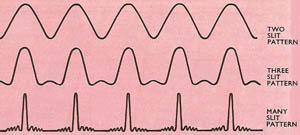
These graphs show the brightness of the fringe on the screen for different numbers of slits, 'downs' being dark, and 'ups' being bright.
Diffraction is the spreading or bending of waves as they pass round the edge of an obstacle or through an opening whose size is roughly the same as the wavelength of the waves. The disturbed waves then interfere (see interference) to produce ripple-like patterns. Diffraction is a property that distinguishes between wavelike and particlelike behavior.
Introduction to diffraction
If part of a beam of light is obstructed it casts shadows. The shadows are sharp if all the rays originate from the same source of light because the unobstructed rays of light appear to travel on in straight lines. The area of shade formed by the obstruction is called the geometrical shadow. It would be rather pointless, for example, to sit in the geometrical shadow of a sunshade if sunlight could bend round the edges by an appreciable amount.
However, although light does take the shortest path in traveling between two points (i.e., light usually travels in straight lines), it is not simply a straight line stream of energy. Light is a kind of wave motion, vibrating up and down and from side to side as it travels forward. This fact makes a difference to the way light casts shadows, although under normal circumstances the difference is too small to be noticed.
Other kinds of waves make areas of 'light' and 'shade' when they encounter an obstacle. Water waves, for example, trying to enter an opening in a harbor wall, do not continue in a straight line and the waves appear to spread out from the opening into the shadow of the harbor walls. This spreading out is called diffraction.
How waves behave at an obstacle
Waves spread out from a point source, such as a disturbance in the surface of water, the crests of the waves forming ever-increasing circles around the disturbance. Putting an obstacle in the path of a water wave stops part of it, but in the places where the wave is not obstructed, the waves appear to start again. Each point in the gap acts as though it were a water disturbance, emitting circular water waves. The shape of the crests of the waves can be found by drawing circles around every point in the gap. (Actually only semicircles need to be drawn since it is safe to assume that the wave does not travel backward.) If the gap is fairly large, adding together the disturbances in the middle of the gap gives a wave of the same shape it had before hitting the obstacle. But at the edges the disturbances spread out into the geometrical shadow.
Light always does this every time it meets an opaque obstacle. If a large amount of the light is allowed to carry on, the diffracted light at the edges will represent only a tiny fraction of the total light, and will be unnoticeable. This is so if light passes through a large hole. However as the size of the hole gets smaller, the proportion of diffracted light increases, since a greater proportion of it is near an edge. A very small hole acts like a single point source of light, with nearly all the light diffracted. The image of a bright and narrow slit has a diffuse area of light around it.
Diffraction of a water wave is easy to see because the waves can be identified at all points of their path. Light waves, on the other hand, can be observed only at the point where they hit something, such as a screen. And the waves vibrate too quickly and travel too fast for individual waves to be seen. The best way of observing the diffraction of light is by making the different rays of light interfere.
Diffraction and interference
Two monochromatic (i.e., single-color) rays of light meeting so that the crests of one coincide with the crests of the other reinforce each other (interfere constructively). If a crest coincides with a trough, however, the two waves cancel (interfere destructively), making a dark spot.
Two narrow slits, illuminated by a monochromatic source so that the crests and troughs of the light going through each slit start off together, give two highly diffracted beams of light. The shadow of the slits is very different from the geometrical shadow. Light spreading out from one slit interferes with light spreading out from the other. The two beams may travel different distances before meeting at the screen, so that the crests of one don't necessarily coincide with the crests of the other. Where crest and crest coincide on the screen is a path of light, and where crest and trough coincide is a patch of darkness. The patches of light and darkness are called interference fringes.
When there are three narrow, equally spaced slits, the interference pattern, instead of getting more complicated, actually becomes more distinct. The extra slit imposes an extra condition for the three beams of light all to interfere constructively at the same place. This has the effect of narrowing down the bright region, at the same time making it brighter, since all the light is concentrated into it.
Adding more and more very narrow, equally spaced slits results in very much brighter and narrower bright fringes. The position of a bright fringe on the screen depends on its color, i.e., wavelength. So different colors are "focused" at different places on the screen. If instead of being illuminated by monochromatic light, the slit is lit by a mixture of all the different colors, or white light, the light is split up into its different colors in much the same way as it is split up by a prism.
Diffraction gratings
When there a tremendous number of slits – typically about 60,000 – the slits form what is called a diffraction grating. The slits can be the spaces between very fine, equally spaced opaque lines on a piece of glass. The lines are ruled with high precision, the 60,000 or so lines on a grating only about three inches wide. The spectra which can be obtained with these gratings are far more precise than the spectra from prisms, so diffraction gratings are often used instead of prisms in spectroscope.
The main difference between gratings and prisms is if, say, it gives a number of complete spectra (from red to violet), where the prism would give only one. Each line corresponds to a bright fringe in the diffraction pattern for two slits. Gratings are ruled from 70 lines per millimeter (for infrared work) to 1800 lines per millimeter (for ultraviolet work).
Fresnel and Fraunhofer diffraction effects
Diffraction effects are classified into either Fresnel or Fraunhofer types. Fresnel diffraction is concerned mainly with what happens to light in the immediate neighborhood of a diffracting object or aperture, so is only of concern when the illumination source is close by. Fraunhofer diffraction is the light-spreading effect of an aperture when the aperture (or object) is lit by plane waves, i.e., waves that effectively come from a source that is infinitely far away. Because of Fraunhofer diffraction, a telescope can never form a perfect image. A point-like source, for example, will be seen as a small disk surrounded by a series of rings; a thin line on a planet will become widened into a band, which decreases in intensity on both sides. The only way to overcome the limitations of diffraction is to use a telescope of larger aperture.
Diffraction-limited
An optical system that is diffraction-limited meets the Rayleigh criterion, or, put another way, can bring light to a focus at the theoretical minimum of the Airy disk. To be diffraction-limited there must be no more than ¼ wavelength of light between wavefronts at the focal point. Modern optical systems can exceed this theoretical design limit, but it remains a standard.
Electron diffraction
Electron diffraction is a method by which the structure of gases and the surface of solids is determined. Just as light waves undergo diffraction when passing through a narrow opening, so also do electrons. The electron wavelength can be adjusted, and the way it behaves after striking the subject material is used to determine the material's crystal structure.