electric space propulsion
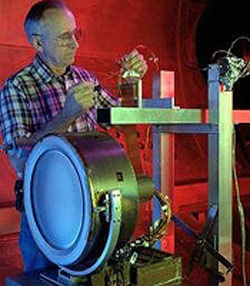
A NASA engineer prepares to test the Deep Space 1 ion engine.
Electric space propulsion is a form of advanced rocket propulsion that uses electrical energy for heating and/or directly ejecting propellant. Electric propulsion (EP) provides much lower thrust levels than conventional chemical propulsion (CP) does, but much higher specific impulse. This means that an EP device must thrust for a longer period to produce a desired change in trajectory or velocity; however, the higher specific impulse enables a spacecraft using EP to carry out a mission with relatively little propellant and, in the case of a deep-space probe, to build up a high final velocity.
The source of the electrical energy for EP is independent of the propellant itself and may be solar (see solar-electric propulsion) or nuclear (see nuclear-electric propulsion). The main components of an EP system are: an energy source, a conversion device (to turn the source energy into electrical energy at an appropriate voltage, frequency, etc.), a propellant system (to store and deliver the propellant), and one or more thrusters to convert the electrical energy into the kinetic energy of the exhaust material.
EP systems fall into three major categories: electrostatic propulsion, electrothermal propulsion, and electromagnetic propulsion. Within each of these categories are several further subdivisions as shown in the table below.
ARCJET | |||
ELECTROTHERMAL | RESISTOJET | ||
MICROWAVE PLASMA | |||
ION | ELECTRON BOMBARDMENT | ||
ELECTRIC | ELECTROSTATIC | PROPULSION | CONTACT ION |
FIELD EMISSION | |||
MAGNETOPLASMA | |||
ELECTROMAGNETIC | PULSED PLASMA | ||
HALL EFFECT |
Of these various types of electric propulsion, several are technologically mature enough to be used on spacecraft, including: the electron bombardment thruster (particularly the xenon ion thruster), the Hall effect thruster, the arcjet, the pulsed plasma thruster, and the resistojet. Other devices such as magnetoplasmadynamic thrusters, contact ion thrusters, and pulsed induction thrusters have not progressed beyond laboratory or theoretical studies. The electron bombardment thruster and the contact ion thruster are both forms of ion propulsion.
History of electric space propulsion
Possibly the first to describe scientifically the possibility of electric space propulsion was Robert Goddard in 1906. Goddard noted from his experiments with discharge tubes that while charged particles were accelerated to great velocities by the electric fields within the tube, the tube walls remained relatively cool. In contrast, no known material could tolerate the temperatures needed to propel gas particles at similar speeds through heating; that is, in a chemical rocket motor. Goddard went on to further conclude that particles accelerated by electrostatic means could be the basis of a high-exhaust velocity propulsion system.
Over the course of six years, Goddard frequently came back to the notion of using electrostatically accelerated particles for spacecraft propulsion. In remarkably prophetic style, he even went so far as to postulate that high-velocity streams of negative [electrons] and positive [ions] particles could be "energized" by solar-electric power supplies to provide thrust for an interplanetary spacecraft. This spacecraft would use chemical propulsion to reach Earth escape velocity but then use EP both to accelerate further and then to decelerate once its target was reached. Goddard postulated that the source of the ions could come from exposing alkaline atoms, such as mercury or cesium, to hot tungsten surfaces. By 1916 Goddard and his students were conducting perhaps the world's first electric propulsion experiments with ion sources. Four years later Goddard devoted passages of his technical reports to his EP experiments.
Hermann Oberth devoted a chapter of his book Possibilities of Space Flight, published in 1929, to various problems of electric propulsion systems and envisioned a scheme that might carry a 150-ton payload. He wrote on EP again in his 1957 book Man into Space. Like Goddard, Oberth felt that the electrostatic acceleration of ions was the key to achieving high exhaust velocity propulsion in space. Oberth describes a possible ion propulsion system in Man into Space as porous plates electrically biased to high voltage that provide a finely distributed "spray" of high-speed charged particles. He states that such an engine could use almost anything as propellant, even the refuse from the spacecraft crew. He notes that the thrust would likely be small (in comparison to the vehicle mass) but steady, and that the engine would operate for a long period of time.
After World War II, Wernher von Braun asked his colleague Ernst Stuhlinger to review Oberth's work in this field. In 1955 Stuhlinger presented a paper at the International Astronautical Congress in Vienna entitled, "Possibilities of Electrical Space Ship Propulsion." During his presentation, Stuhlinger discussed a proposal made by von Braun two years earlier, to use chemical propulsion to send a spaceship to Mars. In von Braun's proposal, Stuhlinger noted that the ratio of takeoff weight to final weight after propellant consumption was 25-to-1. Stuhlinger argued that lighter-weight electric propulsion systems would make such planetary trips more feasible than they were with chemical propulsion. Stuhlinger eventually published a book entitled Ion Propulsion for Space Flight and went on to direct NASA Marshall Space Flight Center's work on arcjet and ion propulsion systems. While he was laying the technical groundwork for ion thruster development between 1947 and 1960, a number of engineers and scientists were writing on the subject.
A crucial contribution to the theory of electric propulsion came in March 1949 with the publication in the Journal of the British Interplanetary Journal of the fourth installment in a series of articles titled "The Atomic Rocket" by L. R. Shepard and A. V. Cleaver. It contained the first quantitative analysis of the feasibility of electrostatic propulsion for interplanetary missions. They considered using a nuclear reactor to heat a propellant such as hydrogen or to provide electric power to an ion thruster. These propulsion approaches are now called nuclear-thermal propulsion (NTP) and nuclear-electric propulsion (NEP), respectively. They concluded that NEP generated much higher exhaust velocities than NTP systems and with considerably less waste heat to reject from the craft. They predicted that exhaust velocities up to 100 kilometers per second would be possible with an NEP system (vs. 10 kilometers per second with a hydrogen-propellant NTP engine). They also suggested configuring the ion exhaust as a beam of parallel streams (as opposed to Oberth's spray concept), concluded that an electron source would be needed to prevent the spacecraft from charging up as positive ions are expelled by the engine, and considered heavy particles to be preferential to light particles to provide high thrust at reduced beam current. However, Shepard and Cleaver concluded that the power requirements were too great for EP to be feasible in the foreseeable future.
Fortunately, the American astrophysicist Lyman Spitzer reached a very different conclusion in a paper he presented just two years later, in 1951. Spitzer played a key role in encouraging the first experiments in EP by not only by his upbeat assessment of its prospects but also by applying the space-charge limited current law, known from the work of C. D. Child and I. Langmuir for about 40 years, to the general design of an ion rocket. He also deserves credit for giving the first quantitative description of a gridded ion thruster, the type now used in the highly successful XIPS. In a series of papers, Spitzer wrote of using fission reactors with turbogenerators to power ion engines for deep space exploration and also concluded that propellant exhaust velocities of 100 km/s would be possible with such a system.
One issue that caused people to question EP's true utility in space travel was the low inherent thrust-to-weight ratios of electric engines. While chemical propulsion systems have thrust-to-weight ratios that often exceed unity (indeed this is needed to get a vehicle off the ground from a vertical launch position), EP systems are expected to have thrust-to-weight values thousands of times smaller than this. In 1953 H. S. Tsien designed trajectories and thrust alignment procedures for low-thrust, EP-propelled spacecraft. Tsien showed that thrust-to-weight ratios as low as 10-5 (1 in 100,000) are sufficient to change the trajectory of a space vehicle over a realistic period of time.
In 1954 Stuhlinger presented the first comprehensive study of the major components associated with an electrically propelled spacecraft. Stuhlinger was the first to show the relationship between the optimum exhaust velocity, the desired delta V, and the specific mass of the power source rigorously. The last quantity, often called alpha (power supply mass divided by power source output power), is a key parameter both for space vehicle design and for identifying the necessary operating characteristics of the EP engine. Stuhlinger also noted that propellants with large mass-to-charge ratios, such as mercury (or xenon) are desirable to minimize the size of the ion engine for a given thrust level.
Over the next few years, a number of people looked at various aspects of EP space travel, including the notion of varying exhaust velocity to optimize the trajectory (J. H. Irving) and deriving an expression for the optimum exhaust velocity as a function of alpha and delta V (D. Langmuir). Project Snooper was started in 1957 by M. I. Willinksky and E. Orr to design a nuclear-electric space vehicle that would carry scientific instruments to deep space. The Snooper vehicle would use chemical propulsion to achieve escape velocity but NEP thereafter. That year also marked a huge transition in the field – from concentrating on overall vehicle attributes to identifying specific technical problems that needed to be addressed. In other words, the year Sputnik I was launched was also the year the scientific community accepted EP as a viable propulsion technology and transitioned from proving EP's worth to solving technical challenges that impeded EP's implementation. These technical challenges included ion beam generation and neutralization, developing lightweight (low alpha) power sources, and intense arc stabilization.
To this end, the Air Force Office of Scientific Research (AFOSR), which is still one of the largest sponsors of EP research in the US, issued the first electric propulsion research grants in 1957. It was also around this time that engineers began to consider other mechanisms, beyond ion acceleration, for electric propulsion. Researchers began looking into systems that use electric arcs to heat gases to high temperature and thus provide thrust by expanding and accelerating the hot gases through a nozzle (as in the case of a chemical rocket), and that use electromagnetic forces to accelerate a highly ionized gas (plasma). By 1959 the Office of Naval Research (ONR), the Army Ballistic Missile Agency (ABMA) and the Advanced Research Projects Agency (ARPA) had all issued research grants in EP. By 1960 almost every large rocket and aircraft firm had an active EP program.
Some of the better-known companies from that time that are still active in the aerospace field today include Lockheed (builder of launch vehicles and communication satellites and the first US company to use EP on a commercial spacecraft, now Lockheed-Martin), Rockwell (builder of the Saturn V launch vehicle and the space shuttle, now part of Boeing), Thiokol Chemical Corporation (builder of the Space Shuttle Solid Rocket Booster, now Morton-Thiokol), General Electric, Aerojet-General (builder of chemical rocket and electric propulsion engines for launch vehicles, space probes and satellites, now called simply Aerojet), and Hughes Aircraft (builder of communication satellites and the first to use ion propulsion in space, now Boeing). In 1958 the National Advisory Committee for Aeronautics (NACA), the predecessor of NASA, established an EP program at the Lewis Flight Laboratory (now the John Glenn Research Center) outside of Cleveland, Ohio. By 1960 EP programs had sprouted up at the Jet Propulsion Laboratory in Pasadena, California, and at the Marshall Space Flight Center in Huntsville, Alabama. By early 1962 the NASA Lewis Research Center was designated as the lead center for Electric Propulsion Research within the agency, a distinction that exists today, some 40 years later! By 1964 NASA Lewis Research Center developed two ion thrusters for testing in space under the SERT I (Space Electric Rocket Test I) program. The thrusters were to run off of batteries mounted on a small capsule that was launched into a 50-minute ballistic flight by a Scout missile. Although one of the thrusters failed to operate, the other was able to answer the question SERT I was developed for: Do ion thrusters operate in space as well as they do in vacuum chambers on the ground? In particular, ion beam neutralization from an auxiliary electron stream in space versus on the ground was of great interest. Over the next few years, a number of space missions would confirm that the performance of EP devices could be measured accurately on the ground.
In 1970 NASA flew SERT II. While SERT I was developed to address ion beam neutralization concerns and whether ion thrusters operate as efficiently in space as they do on the ground, SERT II was designed to demonstrate long-term ion thruster operation in space with a solar power source. On 3 February 1970, the SERT II spacecraft was launched into polar orbit, which permitted the vehicle to receive continuous sunlight (and hence power) during its entire mission. SERT II carried two mercury-propellant ion thrusters, one of which operated for more than five months and the other for nearly three months. Each engine was 15 cm in diameter, consumed 850 watts of power and produced 28 millinewtonsN (~0.006 pound) of thrust. Extended ion thruster restarts were conducted from 1973 to 1981. The engines were started more than 200 times in space and confirmed that ion thruster performance could be both measured on the ground in vacuum chambers and predicted by monitoring grid voltages and beam current. Subsystems of the SERT II spacecraft such as solar arrays were tested through 1991, making the 21-year mission of SERT II one of the longest in history.
Between 1970 and 1990, EP research in the United States focused on mercury- and later xenon-propellant ion thrusters, hydrazine-propellant resistojets and arcjets, hydrogen-propellant arcjets and magnetoplasmadynamic (MPD) thrusters, Teflon-propellant pulsed plasma thrusters (PPTs) and argon-propellant MPD thrusters and Hall thrusters.
The 1990s marked a number of significant events for electric propulsion development worldwide. These events included: Lockheed-Martin launching a communication satellite that used hydrazine-propellant arcjets for stationkeeping (i.e., keeping the satellite in its proper orbit and orientation with respect to Earth); Hughes launching the world's first commercial ion engine on the communications satellite, PanAmSat 5; DS1, NASA's first deep-space mission to use ion propulsion; and The collapse of the Soviet Union, which resulted in an influx of technical information on their EP development activities. The principal EP product to come westward from the Soviet Union was the Hall thruster. Because of its performance characteristics (e.g., exhaust velocity and efficiency) that are ideal for commercial applications in Earth orbit, the Hall thruster has in effect supplanted the hydrazine arcjet (and perhaps to some degree in the future, the ion thruster) as the propulsion system of choice for stationkeeping and orbit raising of large commercial space vehicles.
The group gathered to watch the engine actually operate in a vacuum chamber intended to duplicate the conditions the engine would encounter in space. Less than two months after the demonstration, however, NASA announced the consolidation of its nuclear-electric propulsion programs at Lewis. Nuclear-electric propulsion work at the Marshall Center's Research Projects Division, directed by Stuhlinger, moved from Huntsville to Cleveland. Stuhlinger, though, stayed at NASA/Marshall and worked on other projects. "The urgency of Marshall's work on NASA's lunar and manned space flight programs prompted the decision to employ Stuhlinger's group exclusively on those projects," one agency report stated. The nation was focused on sending a man to the moon using chemically propelled rockets. However, work on EP continued at Lewis.