Mars
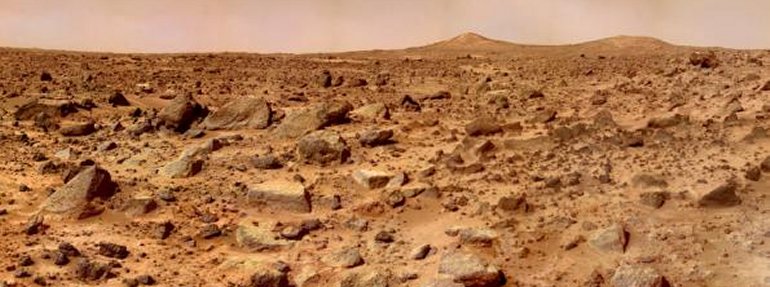
Mars Pathfinder view of the surface of Mars. The scene includes bouldery ridges and hummocks of flood debris that range from a few tens of meters away to the "Twin Peaks" – modest-size hills – about a kilometer (five-eighths of a mile) away.
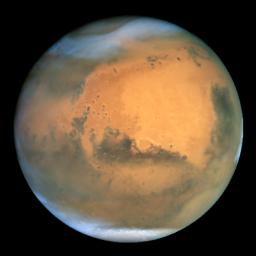
Mars as seen by the Hubble Space Telescope.
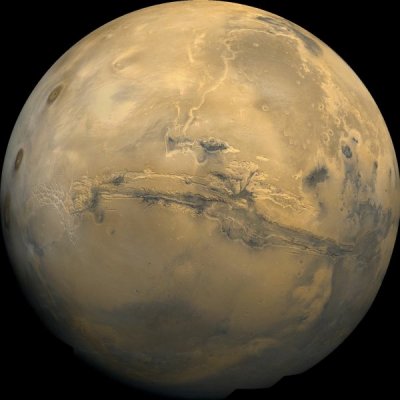
The huge canyon system in the middle of the picture is Valles Marineris.
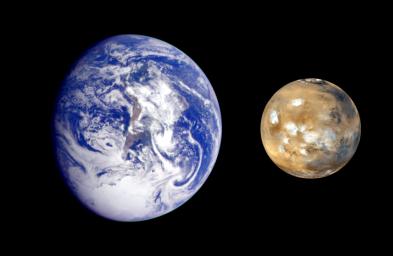
Earth and Mars compared.
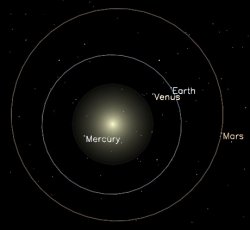
Orbits of the inner planets.
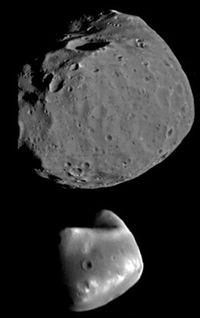
Phobos (top) and Deimos (bottom).
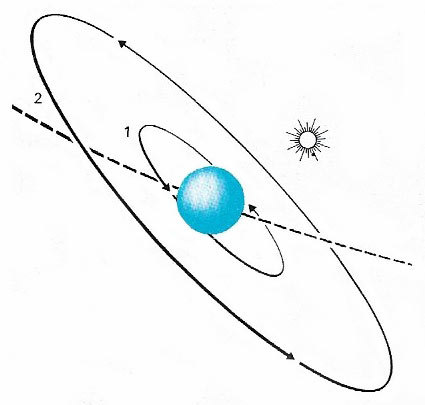
Both the satellites of Mars move in orbits that are practically circular and in the plane of the planet's equator. Phobos (1) is remarkably close to Mars and may approach to within 5,800 km (3,600 mi). It is the only known natural satellite with a revolution period shorter than the rotation of its primary, and as seen from the planet it rises in the west and sets in the east. Deimos (2) is much further out, with a period of 30 h 14 min.
Mars is the fourth planet from the Sun. It is only about half as big as Earth and has only about one-quarter of our planet's surface area. But because Mars doesn't have any oceans or seas, the total area of the martian surface is about equal to that of Earth's dry land.
Mars is named after the Roman God of War, probably because of its red color which suggests blood and battle. It's also commonly known as the Red Planet. Its ruddy color is due to large amounts of red iron oxides (rust), including minerals such as hematite, in the surface rocks and soil.
distance from Sun (mean) | 227.9 million km (141.6 million mi., 1.52 AU) |
distance from Sun (min) | 206.7 million km (128.5 million mi, 1.38 AU) |
distance from Sun (max) | 249.1 million km (154.8 million mi, 1.66 AU) |
equatorial diameter | 6,786 km (4,217 mi) |
equatorial diameter (Earth=1) | 0.532 |
mass (Earth=1) | 0.107 |
density | 3.9 g/cm3 |
axial period | 24.6 hours |
axial inclination | 23.9° |
orbital period | 686.98 days |
orbital inclination | 1.85° |
orbital eccentricity | 0.093 |
atmospheric composition | 95.3% CO2, 2.7% N2, 1.6% Ar, 0.15% O2, 0.03% H2O |
surface temp (mean) | -55°C (-67°F) |
surface temp (equat. summer day) | 27°C (80°F) |
surface temp (polar winter night) | -133°C (-207°F) |
surface gravity (Earth=1) | 0.38 |
escape velocity | 5.0 km/s (18,108 km/h, 11,254 mph) |
albedo | 0.16 |
Orbit
Mars orbits about one and half times farther from the Sun than Earth does, which is the main reason it's so much colder. The orbit of Mars is also noticeably more elliptical than Earth's. This means the amount of solar heat received during the day at any given point on the planet's surface varies widely between when Mars is closest to the Sun (perihelion) and when it's farthest away (aphelion). Big swings in temperature are the result.
Although the yearly average temperature over the whole surface is about –55°C (–67°F), the temperature ranges from as low as –133°C (–207°F) at the winter pole to a surprisingly balmy 27°C (80°F) at the equator on a summer day. These values are generally much lower than would occur on Earth if our planet orbited the Sun at the distance of Mars, due to the relatively feeble greenhouse effect of the thin martian atmosphere.
One martian year equals just under two Earth years because Mars takes 687 days to complete an orbit.
Oppositions
The Earth-Mars distance fluctuates between about 56 million and 400 million kilometers (35 million and 250 million miles), as the two planets swing around their respective orbits. The best times for telescopic viewing are when Mars is in opposition – that is, close to Earth and in the opposite part of the sky from the Sun. These happen every 780 days. But the most favorable oppositions of all, when the two planets approach as close as possible, occur only at intervals of between 15 and 17 years, and always in July, August, or September. On 27 August 2003, the opposition distance of Mars from Earth was the closest it has been for about 60,000 years. Calculations suggest that the last time they were this close together was when Neanderthals roamed the planet, on 12 September 57,617 BC. Other significant encounters happened during the month of August in 1924, 1845, and 1766 but there won't be another close approach for 284 years. Earth has a more-or-less circular orbit while Mars takes a more elliptical path around the star. The two planets travel at different speeds around the Sun, Earth eventually catching and overtaking Mars. This means Mars passes closer to Earth than usual at various times in history, with the 2003 opposition being particularly memorable.
![]() |
Rotation
Mars spins around on its axis once every 24.62 hours. This is the length of the martian day, which is known as a sol. One sol is just 39 minutes longer than an Earth day of 24 hours.
Topography
The two hemispheres of Mars are strikingly different. Most of the southern hemisphere is marked by ancient cratered highlands, similar to those on the Moon. The northern hemisphere, in contrast, is dominated by lava-filled plains that reveal a more complex history of activity and change. At the boundary between the two types of terrain is an abrupt shift height of several km. Scientists still aren't sure about the origin of this sharp boundary and the two contrasting hemispheres. One idea is that Mars suffered a massive impact shortly it formed.
From Earth, the northern plains of Mars look paler than the rest of the planet and were once thought to be continents. Consequently, they were given names such as Utopia Planitia (plain of Utopia) and Arabia Terra (land of Arabia). The darker features in the south were believed to be seas and so were given names such as Mare Erythraeum, Mare Sirenum, and Aurorae Sinus. The largest dark feature on Mars seen from Earth, and the first permanent marking on any planet to be spotted through a telescope, is Syrtis Major.
Surface features
Mars has some outstanding surface features – quite literally. Among its lofty volcanoes is the biggest in the solar system, Olympus Mons. Mars also boasts a collection of volcanoes in the northern Tharsis region so huge that they make a noticeable bump in the planet's roundness; an impact crater in the southern hemisphere, Hellas Planitia, which is more than 6 kilometers deep and 2,000 kilometers wide; and a huge canyon system, Valles Marineris (Valley of the Mariners), long enough to stretch from Los Angeles to New York. Mars may also possess caves.
Argyre Planitia
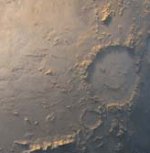
An 800-kilometer-diameter impact basin in the southern hemisphere that is the youngest such structure on the planet, with an age of about 3.5 billion years.
Chryse Planitia
A relatively smooth, circular plain, possibly an ancient impact basin, in the north equatorial region, 1,600 kilometers across and 2.5 kilometers below the mean level of the planet's surface. It seems to have suffered water erosion in the past and was the site of the Viking 1 landing. More here.
Elysium Planitia
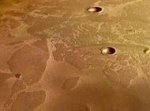
The second largest volcanic region on Mars, after Tharsis Montes. It measures 1,700 by 2,400 kilometers and contains three large volcanoes: Elysium Mons, Hecates Tholus (NE of Elysium Mons), and Albor Tholus (SE of Elysium Mons). More here.
Hellas Planitia
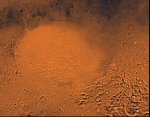
Formerly known simply as 'Hellas,' a near-circular impact basin, some 2,500 kilometers wide and about 6 kilometers deep. It is conspicuous by its color which often appears lighter than surrounding areas due to overhanging mists and cloud. More here.
Ma'adim Vallis
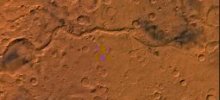
One of the largest valley systems on Mars. Named after the Hebrew for 'Mars,' it is about 860 kilometers long, 8 to 15 kilomeers wide, up to 2,100 meters deep, and located in the highlands of the southern hemisphere. Images sent back by Mars Global Surveyor suggest that Ma'adim Vallis formed some 3.5 billion years ago when a large lake, estimated to have been 1.1 million square kilometers in area and 1,100 meters deep, overflowed a low point in its perimeter.
Olympus Mons
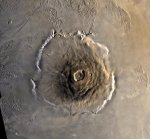
The highest volcano in the solar system – three times higher than Mount Everest. Mons Olympus wouldn't be difficult to climb, though, because it rises very gently from the surrounding plain. The only exception to this is a steep escarpment that borders the summit. A shield volcano, similar to those in the Hawaiian chain but vastly larger, it measures 624 kilometers wide and 25 kilometers high. In the center is a caldera, 80 kilometers wide with multiple circular, overlapping collapse craters created by different volcanic events. The radial features on the slopes of the volcano were formed by overflowing lava and debris. Olympus Mons is found in the Tharsis Montes region near the martian equator. More here.
Syrtis Major
A conspicuous dark, roughly triangular marking, about 1,200 kilometers long and 1,000 kilometers wide, centered at about +10° N, 290° W; it was first noted by Christiaan Huygens in 1659. Formerly known as the Hourglass Sea or the Kaiser Sea, its present name is Greek for 'great sandbank,' which is appropriate since it appears to consist of a large area of wind-blown dust. More here.
Tharsis Montes
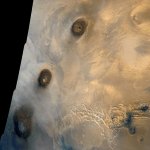
An extensive upland region, from which rise three giant shield volcanoes, Ascraeus Mons, Pavonis Mons, and Arsia Mons, each to about 27 kilometers above the datum level for the planet. Tharsis Montes, also known as the Tharsis Ridge, extends for 2,100 kilometers and varies in height between about 9 kilometers and 11 kilometers above the datum level. More here.
Utopia Planitia
A vast, sparsely-cratered, sloping plain, about 3,200 kilometers across, centered at latitude 48° N, longitude 277°. Viking 2 landed in eastern Utopia Planitia, about 200 km west of the crater Mie. More here.
Valles Marineris
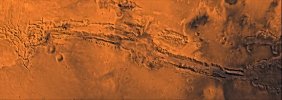
The largest system of canyons in the solar system. Just south of the martian equator, it is about 4,000 kilometers long – as wide as the continental United States. The central individual troughs, generally 50 to 100 kilometers wide, merge into a depression as much as 600 kilometers wide. In places the canyon floor reaches a depth of 10 kilometers – six to seven times deeper than the Grand Canyon. The geologic history of the central canyon system is complex: first the surface collapsed into a few deep depressions that later became filled with layered material, perhaps as lake deposits. Then graben-forming faults cut across some of the older troughs thus widening existing troughs, breaching barriers between troughs, and forming additional ones. At that time the interior deposits were locally bent and tilted, and perhaps water, if still present, spilled out and flowed toward the outflow channels. Huge landslides fell into the voids created by the new grabens. More here
Mars also has permanent ice caps at both poles, which retreat and grow with the seasons. For more about them, see Mars, polar caps.
Geology
Although tectonism has clearly played a major role in the planet's development, it has not involved the lateral movement of sliding plates as on our own world, but instead only vertical movement of hot lava pushing up to the surface. The lack of plate tectonics, as on Mercury and the Moon, has resulted in hot-spots remaining fixed in certain locations beneath the crust. This, along with the lower surface gravity, may account for the Tharsis bulge and its enormous volcanoes. Although there's no evidence of current volcanic activity, observations by Mars Global Surveyor suggest that Mars may have been tectonically active early on, making comparisons with Earth all the more interesting. Lacking plate tectonics today, Mars can't recycle any of the carbon dioxide in its rocks back into its atmosphere and so can't sustain much of a greenhouse effect. Large, but not global, weak magnetic fields exist in various regions of Mars, probably remnants of an earlier global field that has since disappeared.
In many places, there is incontrovertible evidence of erosion and a watery
past, including flood plains and river channels, which tantalize the astrobiologist. Valles Marineris, however,
was created not by running water but by the stretching and cracking of the
crust associated with the formation of the Tharsis bulge.
Interior
Inside, Mars may have a core roughly 1,700 kilometers in radius which the planet's relatively low density suggests contains a higher ratio of sulfur to iron than that in the cores of the other terrestrial worlds. Overlaying this is probably a molten, rocky mantle, somewhat denser than Earth's, topped with a thin crust, which, based on data collected by Mars Global Surveyor, is about 80 kilometers thick in the southern hemisphere and about 35 kilometers thick in the north.
Atmosphere
Mars has a thin atmosphere, which is composed of mainly (95.3%) carbon dioxide. Other gases present include nitrogen (2.7%), argon (1.6%), oxygen (0.15%), water vapor (0.03%), and, intriguingly, methane. The discovery of a bit of methane in the martian atmosphere has excited scientists because they don't know where it has come from. One possibility is geological activity below the surface, another is microbes giving off the gas as part of their metabolism. For more, see Mars, atmosphere.
Water on Mars
How much liquid water existed on Mars in the past, and when? Does liquid water exist on Mars today? These are questions that fascinate scientists, especially astrobiologists, because they have a direct bearing on whether there was once life on Mars and, if so, whether it has survived to the present day.
Images taken by Mars Global Surveyor (MGS) have provided some of the best evidence yet that water still occasionally flows on the Martian surface. Two gullies on the inside of craters, which were originally photographed by MGS in 1999 and 2001, and imaged again in 2004 and 2005, showed changes consistent with water flowing down the crater walls, according to a study published in December 2006.1 The two sites are in the Terra Sirenum and the Centauri Montes regions of southern Mars. In both cases, researchers found bright, light-colored deposits, each several hundred meters long, in the gullies that were not present in the original photos. They concluded that the deposits – possibly mud, salt, or frost – were left there when water recently cascaded through the channels.
![]() |
Enlarged view of a new gully deposit in a crater
in Terra Sirenum.
|
Other scientists challenged this explanation, arguing that the atmosphere of Mars is so thin and the temperature so cold that liquid water couldn't persist at the surface but would rapidly evaporate or freeze. Some of these scientists suggested that the gullies, and others like them discovered by MGS, might have been caused not by water but by liquid carbon dioxide, which has a lower melting point than water. However, the carbon dioxide theory does not bear close scrutiny, for the following reason. The minimum pressure under which a substance can exist as a liquid occurs at the substance's triple point. In the case of carbon dioxide, the triple point corresponds to a temperature of –56.6°C and a pressure of 5.1 atmospheres (518,000 pascals). Now, while Mars does get as cold as (or colder than) –56.6°C, the surface pressure on Mars (or on Earth, for that matter) certainly never reaches 5.1 atmospheres. Thus we can be absolutely certain that carbon dioxide cannot exist as a liquid on or anywhere near the surface on Mars. In contrast, the triple point of water occurs at a mere 611.73 pascals (6.1173 millibars), a pressure that is frequently exceeded on the surface of Mars, in craters and other low-lying areas, according to measurements by Viking and other spacecraft. This fact adds credence to the view that water could remain liquid long enough, after breaking out from an underground source, to carry debris downslope before freezing.
Water in the past on Mars
In previous centuries, astronomers thought that the dark areas they saw on Mars through their telescopes might be seas, and, at the end of the nineteenth century there was much speculation, led by Percival Lowell, about the possibility of Martian canals. By the the early decades of this century, however, it had become clear, because of the very low atmospheric pressure, that little or no surface water could exist on the Red Planet today.
In 1969, Mariner 9 provided the first strong evidence that liquid water had flowed on the surface of Mars in the remote past. Among the thousands of images it sent back from orbit were those of flat-floored channels with eroded banks, sand bars and teardrop-shaped islands, channels with second- and third-order tributary systems, and braided channels which, had they been encountered on Earth, would unhesitatingly have been attributed to episodic flooding. Later probes have added to the evidence of water-carved channels and other features on Mars.
The fact that Mars had flowing water implies that conditions on the planet were once very different than they are today. Yet, curiously, there are no signs that it ever rained on the fourth planet. The water-carved systems on Mars are short and stubby, dividing little upstream, and ending abruptly as if the water had suddenly appeared at that spot rather than having fallen over a large area and become collected. The assumption is that the Martian water erupted from beneath the surface, welling up as a result of volcanic eruptions or asteroid impacts, and then flooded to form channels, and lakes, and perhaps even seas.
An ocean on Mars?
If there were large bodies of water long ago on Mars, where were they, how big were they, and how long did they last? According to one theory, a great ocean once filled the low, flat plains of the northern hemisphere. Today these gently sloping plains are marked by ridges, low hills, and sparsely scattered craters. They are considered to be volcanic in origin and Hesperian (3.5 to 1.8 billion years) in age based on crater counts, but they are much smoother than comparable plains in the south and covered by layers of younger material at least 100 meters thick.
![]() |
What Mars would look like today if it still had the
huge amount of water some researchers believe was present at its formation.
|
In 1989, Timothy Parker of the Jet Propulsion Laboratory and colleagues argued that there was geological evidence of a shorelines.2 The originally proposed shorelines were two discontinuous boundaries between landforms thought to have formed by wave or other water-related processes. Stephen Clifford (Lunar and Planetary Institute) and Parker later refined the outlines and hypothesized that Noachian-aged (3.8 to 3.5 billion years old) bodies of water and ice covered up to one third of the surface of Mars.3 The two most continuous supposed shorelines, called the Arabia and Deuteronilus shorelines, run roughly parallel with the southern boundary of the northern plains. The Arabia shoreline can be traced all around the planet except through the Tharsis region. But its elevation varies by several km, in some places by 11 km – a large range that doesn't fit well with a shoreline interpretation. Features of the proposed shoreline that have been interpreted as formed by wave actions or other marine processes can be equally argued as being formed by mass wasting and volcanic processes. The Deuteronilus boundary is less conspicuous than the Arabia one but has a smaller range in elevations. For nearly half its length the Deuteronilus boundary marks the southern extent of the geologic unit called the Vastitas Borealis Formation. For the rest of its length it is seen only intermittently around clusters of hills or across lava flows. The trouble is, if Deuteronilus is an ancient shoreline, why does it lack key features, such as inward-facing cliffs or channels that end abruptly as they enter a large body of water, that would be expected at the margins of a great sea? The fact is, the shoreline evidence for a northern ocean is not all that convincing.
Evidence from the rovers
![]() |
Spherules found in rock on Meridiani Planum - probably
concretions formed as water evaporated
|
In Mar 2004, NASA scientists involved with the Mars Exploration Rovers put forward evidence that rocks near the Opportunity landing site, on Meridianii Planum, had once been drenched in water – the most convincing and powerful case yet made for long-standing liquid water on the surface of Mars. Several key lines of evidence support this conclusion, including the presence in the rocks of sulfates and small spherules that were probably precipitated out of water, and also the rocks' physical appearance, which includes niches where crystals grew.
Where did all the water go?
If there was once a lot of water on the surface of Mars, where did it all go? One possibility is that some of it evaporated, became water vapor in the atmosphere, and was then lost into space because the gravity pull of the planet couldn't hold on to it. Another possibility is that much of still exists on Mars, as ice. Both the poles of Mars contain water ice. And in 2002, scientists analyzing data from the Mars Observer spacecraft announced that they had found vast quantities of water ice in great swathes of the planet just below the surface.
References
1. Malin, Michael C., Kenneth S. Edgett, Liliya V. Posiolova, Shawn M.
McColley, and Eldar Z. Noe Dobrea. "Present-Day Impact Cratering Rate
and Contemporary Gully Activity on Mars." Science, Vol. 314. no. 5805,
pp. 1573–1577, 2006.
2. Parker, T. J., R. S. Saunders, and D. M. Schneeberger. "Transitional
Morphology in West Deuteronilus Mensae, Mars: Implications for Modification
of the Lowland/Upland Boundary." Icarus, vol. 82, p. 111–145,
1989.
3. Clifford, S. M., and Parker, T. J. "The evolution of the Martian hydrosphere:
Implications for the fate of a primordial ocean and the current state
of the northern plains." Icarus, vol. 154: 40–79, 2001.
Life on Mars
People have long wondered if there might be life on the fourth planet. For more about this whole subject, see Mars, life. In the second half of the nineteenth century, speculation about Martians stepped up a gear when some observers reported seeing channels, or what came to be called "canals," on the surface. The seasonal waxing and waning of the martian polar caps was seen by early observers to be accompanied by surface changes at lower latitudes. The darkening of certain regions, following each spring thaw, prompted theories of inundation by floodwater and the growth of vast tracts of plant life.
Ever since then, questions about the existence of life and water on Mars have been entwined. Observations by spacecraft have shown that, in the remote past, conditions on the fourth planet were friendlier to life as we know it than they are today. Mars has been through periods when it was warmer and wetter than it is now. However, we still don't know if life managed to develop under those milder conditions. And, if it did, whether it survived to the present day.
Past conditions on Mars
Early in the 4.6-billion-year history of the solar system, the conditions on Earth and Mars were almost certainly much more similar than they are now. On both planets the primitive atmosphere is thought to have originated in large part from gases, mainly water vapor, carbon dioxide, and nitrogen, vented from volcanoes. In Earth's case, far more water was released than the atmosphere could hold and the bulk of it condensed to form oceans which remain to this day. Evidence suggests that an ocean also formed on Mars, covering, according to some estimates, one-third of the planet's surface to an average depth of 1,700 meters (5,500 feet). Early on, large amounts of carbon dioxide in the martian atmosphere probably led to a vigorous greenhouse effect which kept the average temperature above 0°C. This fact, together with the substantial atmospheric pressure (perhaps similar to that presently found on Earth), would have enabled surface water to remain as a liquid. But whereas on Earth, ongoing plate tectonics have ensured that volcanoes and their release of fresh carbon dioxide play a crucial role in maintaining our planet's equable climate, on Mars the crust appears to consist of a single plate. Owing to its small size, Mars does not have enough internal heat to drive a significant level of tectonic and volcanic activity. Perhaps it did for a while when it was young. But after the martian volcanoes fell silent no new carbon dioxide would have been pumped into the atmosphere, and that which was already there would have been gradually depleted. As happens all the time on Earth, large quantities of atmospheric carbon dioxide were probably taken up by liquid water on the surface and converted into carbonates. Ironically, therefore, the martian ocean and rivers might well have been instrumental in their own demise for, as they removed more and more carbon dioxide, they would have brought about a lowering of the atmospheric pressure and a reduction in the greenhouse effect which had previously maintained the surface temperature above the freezing point of water.
Putting an accurate timescale on these dramatic changes in climate is not easy and researchers continue to argue over when and for how long Mars was warm and wet. The original, volcanically-vented martian atmosphere was probably in place around 4 billion years ago, toward the end of the period of heavy primeval bombardment. Judging by the structure of the valley systems and the extent to which some craters from the early bombardment phase have apparently been eroded by running water, a strong greenhouse effect must have persisted for about 500 million years. Even after this, however, some surface water presumably remained for a long time, continuing to remove carbon dioxide, in order that the atmosphere should have thinned out to its present extent. According to one estimate, the last dregs of the once great ocean on Mars disappeared some 3 billion years ago.
Whether Mars has had an occasional wet spell since is a matter of controversy. It may be that Mars experiences periodic ice ages as does Earth and that, at present, it is in the midst of a prolonged cold snap. There could be a number of reasons for this. Variations in the tilt of the martian rotation axis, for example, are much more pronounced than in the case of Earth due to the gravitational influence of Jupiter. Another possible source of climate change could be fluctuations in the amount of solar energy reflected back into space by the martian polar caps. Wind-borne dust, which becomes trapped in the ice, causes the caps to darken and brighten on a seasonal basis. A gradual long-term reduction in the reflectivity of the poles could cause them to warm up and melt, releasing sufficient carbon dioxide to allow liquid water to exist again on the surface. (Some means of bringing this about artificially might one day be at the heart of a scheme to terraform the Red Planet.) Finally, it is conceivable that variations in the luminosity of the Sun have had their effect on Mars. Some of the channels, presumed once to have contained water, are pock-marked by occasional impact craters, the frequency of which provides a rough measure of the age of these particular channels – about 1 billion years, according to some estimates. This coincides more or less with the most recent era of high global temperatures on Earth, suggesting a common underlying process at work – a possible solar link.
In October 1999, researchers announced that, based on high-resolution images from Mars Global Surveyor, no ancient shorelines could be discerned and that, as a result, the case for past oceans on Mars had been weakened.
Amazonian epoch
The Amazonian epoch is the most recent of the three broad time periods into which the geologic history of Mars has been divided. The Amazonian epoch extends from about 1.8 billion years ago to the present day. From oldest to youngest the martian epochs are the Noachian, Hesperian, and Amazonian (named after places on Mars). They are defined by the number of impact craters on the ground – older surfaces having more craters. The precise timing of the epochs is not known because different models exist of the rates of meteorite falls on Mars.
Hesperian epoch
The Hesperian epoch is the middle of three broad time periods into which the geologic history of Mars has been divided. The Hesperian epoch extends from about 3.5 to about 1.8 billion years ago.
Noachian epoch
The Noachian epoch is the oldest of the three broad time periods into which the geologic history of Mars has been divided. The Noachian epoch extends from the birth of Mars to between 3.8 and 3.5 billion years ago.
Moons
There had been early (groundless) speculation about the existence of two martian satellites (see Swift, Jonathan and the moons of Mars) but it was not until 1877 that diminutive Phobos and Deimos were discovered by Asaph Hall at the US Naval Observatory.1 Working at the same site, Bevan Sharpless concluded in 1944, after analyzing measurements made since Hall's discovery, that the orbit of Phobos was decaying.2 Rapidly, his data suggested, the little moon was spiraling on a collision course with Mars.
For a number of years, this result remained a minor curiosity. Then, in 1959, Soviet astrophysicist Iosef Shklovskii took up the challenge to explain why the orbit of Phobos was shrinking. He looked at the possibility that the thin upper regions of the martian atmosphere might be gradually slowing Phobos down, but decided this effect would be too small to account for the results Sharpless had obtained. Among the alternatives he considered were the influence of the Sun, a tidal interaction with the gravity of Mars, and the effect of a hypothetical martian magnetic field. However, none of these seemed able to provide a satisfactory mechanism. So Shklovskii went back to the idea that atmospheric drag might somehow be involved and came up with an audacious suggestion. What, he asked, if Phobos were not an ordinary moon? What if its average density were only a thousandth that of water, so that the thin outer atmosphere of Mars could act as an effective brake and destabilize the little moon's orbit? What, in fact, if Phobos were hollow? A hollow object 24 kilometers (15 miles) across could not possibly be natural. It would have to be the product of a technology well in advance of our own, surpassing even that required to build the great network of waterways and pumping stations envisaged by Lowell. In Intelligent Life in the Universe, coauthored with Carl Sagan, Shklovskii writes:3
Since Mars does not have a large natural satellite such as our moon, the construction of large, artificial satellites would be of relatively greater importance to an advanced martian civilization in its expansion into space... The launching of massive satellites from Mars would be a somewhat easier task than from Earth, because of the lower martian gravity.
Shklovskii thought it unlikely that the creators of Phobos were still alive:
Perhaps Phobos was launched into orbit in the heyday of a technical civilization on Mars, some hundreds of millions of years ago.
But the idea of advanced extant martian life did not seem beyond belief to some others, including Frank Salisbury, Professor of Plant Physiology at Colorado State University. He pointed out that neither of the martian moons had been spotted in 1862, when Mars was closer than in Hall's discovery year of 1877 and when large instruments were trained upon it:
Should we attribute the failure of 1862 to imperfections in the existing telescopes, or may we imagine that the satellites were launched into orbit between 1862 and 1877?
Sadly, close-up photographs of Phobos taken by the Viking orbiters and other probes, have revealed a more prosaic truth. There is nothing suggestive of artifice about this rocky, pock-marked, potato-shaped moon. In all likelihood it was once, like Deimos, an asteroid which, during its solar peregrinations, strayed too far into the gravitational domain of Mars and became ensnared. Measurements made during the favorable opposition of Mars in 1988 confirmed that the orbit of Phobos is unstable. However, its rate of decay is only about half that claimed by Sharpless so that it can be accounted for in terms of frictional forces acting on a natural, solid body. Each year the little moon draws 2.5 cm closer to its parent planet. Phobos never came from the surface Mars. But it will eventually end up there, blasting a crater roughly 500 kilometers (300 miles) across some time within the next 40 million years.
References
1. Gingerich, O. "The Satellites of Mars: Prediction and Discovery," Journal of the History of Astronomy, 1, 109 (1970).
2. Sharpless, B. P. "Secular Accelerations in the Longitudes of the Satellites
of Mars," Astronomical Journal, 51, 185 (1945).
3. Shklovskii, I. S., and Sagan, Carl. Intelligent Life in the Universe.
New York: Dell (1966).