antimatter propulsion
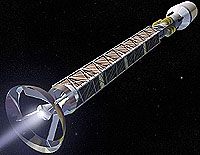
Figure 1. Hypothetical starship powered by antimatter.
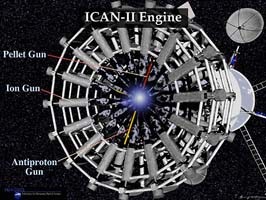
Figure 2. Diagram of Penn State's proposed ICAN-II (ion compressed antimatter nuclear II) spacecraft.
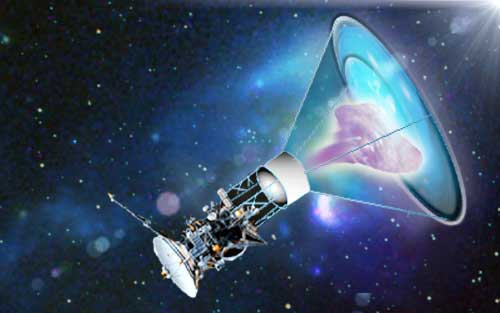
Figure 3. Antimatter-driven sail for a Kuiper-belt mission. Art: Hbar Technologies, LLC/Elizabeth Lagana
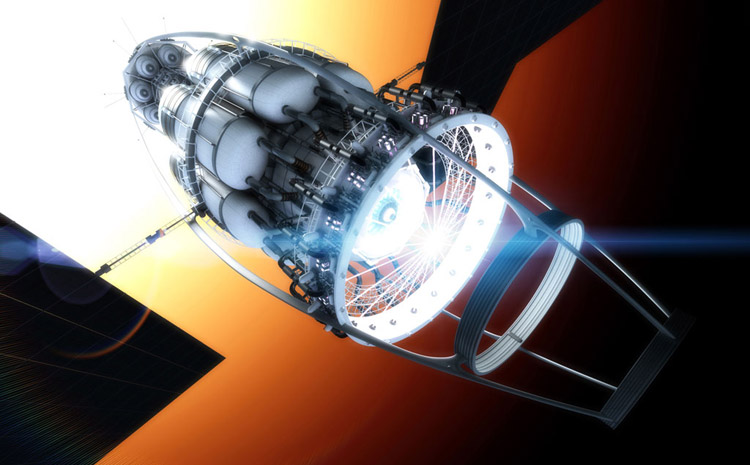
Figure 4. VARIES concept artwork. Credit and ©: Adrian Mann.
Devotees of Star Trek will need no reminding that the starships Enterprise and Voyager are powered by engines that utilize antimatter. Far from being fictional, the idea of propelling spacecraft by the annihilation of matter and antimatter is being actively investigated at NASA's Marshall Space Flight Center, Pennsylvania State University, and elsewhere. The principle is simple: an equal mixture of matter and antimatter provides the highest energy density of any known propellant. Whereas the most efficient chemical reactions produce about 1 × 107 joules(J)/kg, nuclear fission 8 × 1013 J/kg, and nuclear fusion 3 × 1014 J/kg, the complete annihilation of matter and antimatter, according to Einstein's mass-energy relationship (E = mc2), yields 9 × 1016 J/kg. In other words, kilogram for kilogram, matter-antimatter annihilation releases about ten billion times more energy than the hydrogen/oxygen mixture that powers the Space Shuttle Main Engines and 300 times more than the fusion reactions at the Sun's core.
However, there are several (major!) technical hurdles to be overcome before an antimatter rocket can be built. The first is that antimatter does not exist in significant amounts in nature – at least, not anywhere near the solar system. It has to be manufactured. Currently the only way to do this is by energetic collisions in giant particle accelerators, such as those at FermiLab, near Chicago, and at CERN, in Switzerland. The process typically involves accelerating protons to almost the speed of light and then slamming them into a target made of a metal such as tungsten. The fast-moving protons are slowed or stopped by collisions with the nuclei of the target atoms, and the protons' kinetic energy converted into matter in the form of various subatomic particles, some of which are antiprotons – the simplest form of antimatter. So efficient is matter-antimatter annihilation that 71 milligrams of antimatter would produce as much energy as that stored by all the fuel in the Space Shuttle External Tank. Unfortunately, the annual amount of antimatter (in the form of antiprotons) presently produced at Fermilab and CERN is only 1–10 nanograms (a nanogram is a million times smaller than a milligram).1 On top of this production shortfall, there is the problem of storage. Antimatter cannot be kept in a normal container because it will annihilate instantly on coming into contact with the container's walls. One solution is the Penning Trap – a supercold, evacuated electromagnetic bottle in which charged particles of antimatter can be suspended. Antielectrons, or positrons, are difficult to store in this way, so antiprotons are stored instead. Penn State and NASA scientists have already built such a device capable of holding 10 million antiprotons for a week. Now they are developing a Penning Trap with a capacity 100 times greater.2 At the same time, FermiLab is installing new equipment that will boost its production of antimatter by a factor of 10–100.
A spacecraft propulsion system that works by expelling the products of direct one-to-one annihilation of protons and antiprotons – a so-called beamed core engine – would need 1–1,000 grams of antimatter for an interplanetary or interstellar journey.3 Even with the improved antiproton production and storage capacities expected soon, this amount of antimatter is beyond our reach. However, the antimatter group at Penn State has proposed a highly efficient space propulsion system that would need only a tiny fraction of the antimatter consumed by a beamed core engine. It would work by a process called antiproton-catalyzed microfission (ACMF).4 Whereas conventional nuclear fission can only transfer heat energy from a uranium core to surrounding chemical propellant, ACMF permits all energy from fission reactions to be used for propulsion. The result is a more efficient engine that could be used for interplanetary manned missions. The ICAN-II (ion compressed antimatter nuclear II) spacecraft designed at Penn State (Figure 2) would use the ACMF engine and only 140 nanograms of antimatter for a manned 30-day crossing to Mars.
A follow-up to ACMF and ICAN is a spacecraft propelled by AIM (antiproton initiated microfission/fusion) in which a small concentration of antimatter and fissionable material would be used to spark a microfusion reaction with nearby material. Using 30–130 micrograms of antimatter, an unmanned AIM-powered probe – AIMStar – would be able travel to the Oort Cloud in 50 years, while a greater supply of antiprotons might bring Alpha Centauri within reach.
Antimatter-driven sail
An antimatter-driven sail is a type of space sail in which the products of a matter-antimatter reaction are allowed to strike the inside surface of the sail and thus provide thrust (Figure 3). Evaluation of such a system has been carried out by Hbar Technologies with sponsorship by the NASA Institute for Advanced Concepts (NIAC), an institute of the Universities Space Research Association. The ultimate goal of the project is to identify the technological hurdles of dispatching a lightweight instrument package to another stellar system. First phase of work is foreseen as a "less demanding" mission: Sending a probe to the Kuiper belt in a decade's time. The company's work to date suggests that a small instrument payload could be sent out to a distance of 250 astronomical units (AU) from the Sun in 10 years using 30 milligrams of anti-hydrogen. Preliminary calculations also indicate that a similar probe could be sent to the next star, Alpha Centauri, actually a triple star system, in 40 years using grams of antimatter.
VARIES
VARIES (Vacuum to Antimatter Rocket Interstellar Explorer System) is an advanced space propulsion concept (Figure 4) proposed by Richard Obousy, cofounder and president of Icarus Interstellar Inc., that would use large solar arrays to generate power for extremely high-intensity lasers.5 These lasers would take advantage of a quantum effect known as Schwinger pair production to create particles of antimatter, which could be stored and used as fuel. This in-situ refueling process would be used at the vessel's destination to generate fuel for the return journey.
Schwinger pair production
A vacuum, which in classical physics is simply empty space, devoid of matter and energy, in quantum mechanics is shown to be replete with vast amounts of energy. If these immense, hidden reserves of energy could be tapped, they would become available for challenging projects such as high-speed interstellar travel.
Following the discovery by Paul Dirac that a relativistic description of electrons implied the existence of an antiparticle to the electron, called the positron, the US physicist Julian Schwinger showed that, in theory, a sufficiently strong electric field would create electron-positron pairs out of the vacuum of space itself. In the VARIES concept, a starship would employ solar panels an immensely powerful laser to generate its own antimatter fuel using the light from an alien star, once it had arrived at its destination. A sufficient amount of antimatter having been created and stored, the VARIES starship would then be adequately fueled to begin its return trip to Earth.
References
1. Schmidt, G., Gerrish, H., Martin, J. J. "Antimatter Production for
Near-term Propulsion Applications," 1999 Joint Propulsion Conference.
2. Smith, G. A., et al. "Antiproton Production and Trapping for Space
Propulsion Applications," unpublished paper. Read on-line here.
3. Forward, R. L. "Antiproton Annihilation Propulsion," Journal of
Propulsion, 1 (5), 370-74 (1985).
4. Smith, G. A., Gaidos, G., Lewis, R. A., Meyer, K., and Schmid, T.
"Aimstar: Antimatter Initiated Microfusion for Precursor Interstellar
Missions," Acta Astronautica, 44 183-86 (1999).
5. Obousy, R. K. "Vacuum to Antimatter Rocket Interstellar Explorer
System." JBIS 64, No.11/12, 378–386 (2011).