lithium and the 'lithium problem'
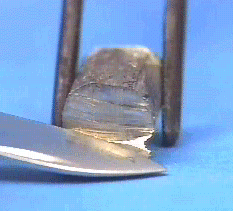
A sample of lithium freshly cut.
Lithium is a soft, silvery, reactive metallic element. It is the lightest of all metals and the least reactive of the alkali metals, which occur in group I of the periodic table.
Discovery
Lithium was discovered by Johan A. Arfvedson in Stockholm in 1817. It was first isolated by W. T. Brande and Humphrey Davy in the 19th century, but was not commercially produced until 1923. Its name comes from the Greek lithos meaning 'stone.'
Sources of lithium
Some lithium is recovered from the mineral spodumene. Commercial quantities of spodumene are found in a special igneous rock deposits known as pegmatites. In pegmatites, the liquid rock (magma) cools so slowly that crystals have time to grow very large. The largest spodumene crystal ever found was in a pegmatite formation in South Dakota.
Most lithium is recovered from brine, or water with a high concentration of lithium carbonate. Brines trapped in the Earth's crust (called subsurface brines) are the major source material for lithium carbonate. These sources are less expensive to mine than from rock such as spodumene, petalite, and other lithium-bearing minerals.
Lithium is made by electrolysis of fused lithium chloride.
Properties of lithium | |
---|---|
atomic number | 3 |
relative atomic mass | 6.941 |
electron configuration | 1s22s1 |
atomic radius | 152 pm |
relative density | 0.534 (at 20°C) |
melting point | 180.5°C; (357.0°F;) |
boiling point | 1,342°C; (2,448°F;) |
Uses of lithium
Lithium is used as a heat-transfer medium, because of its high specific heat, and in various alloys, ceramics, and optical forms of glass. Its two stable isotopes are the rarer 6Li, with three protons and three neutrons, and the more common 7Li, with three protons and four neutrons. 6Li is important in thermonuclear processes. Lithium stearate is an additive to lubricating greases.
The 'lithium problem'
Lithium is one of the few elements, along with hydrogen and helium, that were formed in the immediate aftermath of the Big Bang. The trouble is the amount of lithium observed in the universe is three to four times lower than what our physics of the Big Bang predicts. Several theories have been put forward to explain this discrepancy.
It may be that our understanding of what happened in the period immediately after the Big Bang is wrong or incomplete. For example, it's been suggested that dark matter may have played a role in depleting the cosmic supply of lithium right from the start. Another possibility is that there's some process going on in interstellar space, yet to be fathomed, which, over billions of years, has run down the lithium stock. Or it may be that the missing lithium is hiding deep within stars, having been processed and moved inward in some way not yet understood.
Unfortunately, a study published in 2012 has made the lithium problem even more acute because it predicts that some small black holes – those weighing in at about 5 solar masses – should act as lithium factories, so there should be even more of the stuff around than what we're seeing.[1] In the accretion disk of such black holes, the study finds, the temperature should be just right to trigger nuclear fusion reactions that would produce large amounts of lithium – in total matching that supposed to have been manufactured shortly after the Big Bang.
Another piece of research, also published in 2012, looked at the lithium content of gas clouds in the Small Magellanic Cloud and again confirmed that we have a missing lithium problem.[2] Although the gas has nearly as much lithium as Big Bang models they ought to contain very much more considering how much lithium has been manufactured inside stars since the Big Bang and then shot into space during supernova explosions.
Lithium-rich star
A lithium-rich star is a star with an anomalously high abundance of lithium in its upper layers as revealed by a strong absorption line of neutral lithium (Li I) at 6708 Å. Most lithium stars are carbon stars, but lithium enrichment has also found in some normal late-type giants and T Tauri stars. These objects present astronomers with a puzzle because stars destroy most of their lithium soon after formation. Lithium is consumed at nuclear fusion temperatures and not normally remade. For example, the Sun has a lithium abundance about 100 times less than that of the interstellar medium (ISM). High amounts of lithium would certainly not be expected in aging stars such as red giants. Yet some of these evolved suns show lithium in quantities far higher than that of the Sun, and, in extreme cases, as high as that of the ISM. One possible explanation is the recent infall of a large planet or brown dwarf. Such planet swallowing would provide a sudden fresh supply of lithium (which might last 100,000 years or so) and show up as an excess in the star's spectrum. Although this idea, made all the more likely by discoveries of massive planets in tiny orbits around their host stars, works well in most cases, it struggles when faced with the most extreme examples of lithium overabundance found in objects such as the subgiant halo star BD+23 3912. One way a red giant can make fresh helium is by the transport of 7Be from the core to the envelope where it can be converted to 7Li by electron capture. The only known process for manufacturing 6Li is by spallation involving cosmic rays. But these mechanisms, too, are hard pressed to account for the greatest cases of lithium excess. Possibly a combination of factors is at work or there are lithium-generating mechanisms inside stars that are as yet poorly understood.
Lithium depletion boundary
Lithium depletion boundary (LDB) is an age-dating method proposed for open clusters based on determination of the lithium abundances of cluster members whose masses are near the hydrogen burning mass limit. The idea behind this method is that for stars near the substellar mass limit, the age at which stars become hot enough in their cores to burn lithium is a sensitive function of mass. Furthermore, it is argued that the physics required to predict the location of the LDB as a function of age is well understood and not subject to much uncertainty. In the mass range of interest, stars are fully convective, and the core lithium abundance will be directly reflected in the surface lithium abundance, which can be determined from measurements of the 6708 Å Li I doublet.
References
1. Iocco, F. and Pato, M. "Lithium synthesis in microquasar accretion." Physical Review Letters, Vol. 109, July 13, 2012.
2. Howk, J. C., Lehner, N., Fields, B. D., and Mathews, G. J. "Observation
of interstellar lithium in the low-metallicity Small Magellanic Cloud." Nature, 489, 121–123 (2012).